The U.S. Department of Energy’s Industrial Technologies Program recently received the following question: “My plant is looking for recommendations for achieving boiler combustion control more efficiently. Do you have any suggestions?” A DOE Energy Expert provided the answers.
When investigating steam systems, the boiler is one of the primary targets for energy-efficiency improvement. There are many tools used in the evaluation and management of boiler performance. One of the most useful tools is boiler efficiency. Boiler efficiency describes the fraction of fuel energy that is converted into useful steam energy.
Of course, the fuel input energy that is not converted into useful steam energy represents the losses of the boiler operation. Boiler investigations generally examine the losses by identifying the avenues of loss, measuring the individual loss and developing a strategy for loss reduction.
There are many avenues of loss encountered in boiler operations. Typically, the dominant loss is associated with energy leaving the boiler with combustion gases. The temperature of exhaust gases is an indication of their energy content.
Ensuring that the heat-transfer surfaces of the boiler are clean is a major point of focus for managing the thermal energy in exhaust gases. Energy can be recovered from exhaust gases by transferring thermal energy from the high-temperature gases to boiler feedwater, or to the combustion air entering the boiler.
Another aspect of exhaust-gas energy management, which is the focus here, is combustion management. It should be noted that the temperature- and combustion-related attributes of exhaust gases are interrelated – they combine to represent the stack loss of the boiler. Again, this is typically the dominant loss for the boiler.
Stack loss is dependent on the operating characteristics of the boiler, the equipment installed and the type of fuel burned in the boiler. Stack loss generally ranges from as much as 30 percent for a green-wood-fired boiler, to 18 percent for a typical natural-gas-fired boiler, to 12 percent for an oil-fired boiler, to as low as 9 percent for a coal-fired boiler. It must be pointed out that the stack-loss range is wide for any given fuel.
To address your question, we will examine the combustion of a simple fuel – methane (CH4). The chemical equation for the reaction of methane with oxygen (O2) is presented below:
CH4 + 2O2 → CO2 + 2H2O
In a perfect world, methane will react with oxygen to release energy and form carbon dioxide and water. In this perfect arrangement, each molecule of fuel would find the exact amount of oxygen needed to cause complete combustion. In the case of methane, one molecule of methane must find two molecules of oxygen in order to produce a complete reaction.
In the real world, however, the combustion process does not proceed in a perfect manner. A fuel molecule may encounter less oxygen than is required for complete combustion. The result will be partial combustion; the exhaust gases will then contain some unreacted fuel and some partially reacted fuel. Generally, these unburned fuel components are in the form of carbon monoxide (CO), hydrogen (H2) and other fuel components that may include the fully unreacted fuel source, which in this case is methane.
When unburned fuel is found to be part of the combustion products, a portion of the fuel that was purchased is consequently discharged from the system, unused. It is also important to note that unburned fuel can accumulate to a point where a safety hazard could result. Unburned fuel can burn in a part of the boiler not designed for combustion – under certain conditions, the materials can even explode. Additionally, these chemicals are typically toxic in nature, presenting health and environmental hazards.
CH4 + 2O2 → αCO2 + βH2O + γCO + δH2 + εCH4 + ζO2
Energy Release
Unburned fuel presents negative safety, health, environmental and economic impacts to the boiler operations. As a result, it is imperative to manage the combustion process in order to maintain these components at minimum levels. Fortunately, the complex interrelations of the combustion process can be managed with two fairly simple principles.
The first principle of combustion management is based in the fact that unreacted fuel components are undesirable in the exhaust gases of the boiler, but the presence of unreacted oxygen presents minimal safety, health and environmental concerns. Furthermore, as long as the burner is appropriately mixing the oxygen and fuel, the presence of extra oxygen in the combustion zone essentially ensures that all of the fuel will react completely. The fan energy required to move ambient air into the combustion zone is generally minimal when compared to the cost of fuel.
Combustion management principle No. 1, stated simply, is provide more oxygen than you theoretically need to ensure that all of the fuel burns up. As a result, when combustion management principle No. 1 is applied to the example chemical reaction, rather than two molecules of oxygen being supplied for every one molecule of methane, each molecule of methane may be provided three or four molecules of oxygen.
This will ensure that all of the fuel is burned. The extra oxygen that is added to the combustion zone, however, enters at ambient temperature and it exits the boiler at flue-gas temperature. The flue-gas temperature of a typical boiler could be anywhere in the range of 300 to 500 degrees Fahrenheit range. As a result, the extra oxygen could have entered the boiler at 70 degrees F and exit at 400 F.
The extra oxygen reached this temperature by receiving fuel energy – in other words, fuel was purchased to heat the extra oxygen. Additionally, ambient air contains almost four molecules of nitrogen (3.76 to be exact) for every one molecule of oxygen. As a result, every amount of excess air brings with it a huge amount of nitrogen. This nitrogen enters at ambient temperature and receives fuel energy to exit the boiler at flue-gas temperature.
This brings us to combustion management principle No. 2 – do not use too much oxygen. In other words, combustion management requires that extra oxygen be provided to the combustion zone in order to ensure that all of the fuel is reacted; however, the amount of extra oxygen must be minimized to reduce energy loss. The critical measurements required to manage the combustion loss are the flue-gas oxygen content and the flue-gas combustibles concentration.
The flue-gas oxygen content is measured to allow the combustion air flow to be modified to meet a set point. Combustibles concentrations are measured to identify the minimum practical oxygen concentration. It should be noted that combustibles concentrations can be elevated even though excess oxygen is provided to the combustion zone. This situation is typically indicative of a burner failure.
A primary function of a burner is to mix the air and fuel thoroughly to ensure complete combustion. If a burner component has failed or is improperly adjusted, the mixing process can be ineffective. This can result in unburned fuel and excess oxygen. Combustibles concentration values vary based on fuel type, operating conditions, and burners.
A typical natural-gas-fired boiler will operate with combustibles concentrations less than 50 parts per million (ppm), while a coal-fired boiler may operate with combustibles concentrations greater than 200 ppm. Generally, combustibles concentrations less than 100 ppm are considered negligible in terms of efficiency impact. Baseline combustibles concentrations should be established for each boiler.
The principles and measurements used in combustion management outlined above indicate that combustion control should incorporate flue-gas oxygen measurement, combustibles measurement and active control of combustion airflow. Many boilers, however, do not incorporate these factors into the control process. Common boiler-control strategies are based primarily on steam-pressure control. As the process steam demand increases, the steam pressure at the boiler deceases.
The boiler steam-production controller will measure this decrease in pressure and will increase fuel flow to the boiler. In the case of a natural-gas-fired boiler, the controller will proportionally open the fuel control valve. As the fuel flow is increased, the combustion air flow must also increase in order to maintain safe and efficient combustion.
A very common and simple method of accomplishing the control of combustion air flow is to mechanically link the air-flow control device to the fuel-flow control device. This is commonly called positioning control because the air-flow-control device will have a position that is based solely on the position of the fuel-flow-control device. It should be noted that this control does not incorporate any active oxygen or combustibles measurements.
Oxygen and combustibles measurements are only taken to establish the position relationship between the fuel controller and the air controller. After the position relationship is established, oxygen and combustibles measurements cease.
Tuning the boiler is the act of re-establishing the position relationship between the air and fuel. This tuning activity is completed in the same manner that the original air-fuel control point positions were established. The boiler is operated steadily at discrete fuel input positions and the airflow control device position is redefined.
The boiler will be operated at discrete loads throughout the operating range of the unit. While the fuel-flow controller is 100 percent open, for example, the position of the air-flow controller is adjusted until an appropriate flue-gas oxygen content is attained. Combustibles concentrations also should be measured to ensure proper burner operation.
The position relationship exercise is repeated over the operating range of the boiler (95 percent load, 90 percent load, down to minimum load). This retuning activity should be completed frequently to ensure safe and efficient boiler operation.
It should be noted that when positioning control is used, the oxygen content cannot be minimized because of many factors. One factor influencing the airflow controller position is ambient temperature. Ambient temperature is a concern because the combustion air fan is basically a constant volume-flow device (for a given controller set point).
If the position relationship is established for a relatively cool inlet-air temperature, the mass flow of air into the combustion zone could be dangerously low as the inlet air temperature increases. As a result, positioning control can only attain moderate efficiency.
Combustion control can be improved through the use of an automatic oxygen trim system. This type of system continuously measures flue-gas oxygen content and adjusts the combustion air flow to maintain a set point. This type of control can be more precise and efficient than positioning control because it continually tunes the air-fuel relationship. Combustibles measurement and control can be added to allow the oxygen set point to be minimized.
These control strategies take many forms, and there are many variations of each; this description only outlines the primary composition of the control. It should be noted that most boilers require higher flue-gas oxygen content at lower loads. This generally results from the fact that mixing is compromised in the burner at low loads.
Additionally, flue-gas oxygen content targets will be influenced by additional environmental controls, such as nitrogen oxide control. When a boiler is equipped with nitrogen oxide control, the minimum oxygen concentrations are somewhat higher than in boilers without the nitrogen oxide control.
This article was provided by the U.S. Department of Energy’s Industrial Technologies Program and first appeared in an issue of its Energy Matters newsletter. Ask the Energy Expert is an ongoing column designed to provide information and solutions for industry’s most pressing questions. This issue’s Energy Expert is Dr. Greg Harrell, PhD, PE, of Energy Management Services in Jefferson City, Tenn.
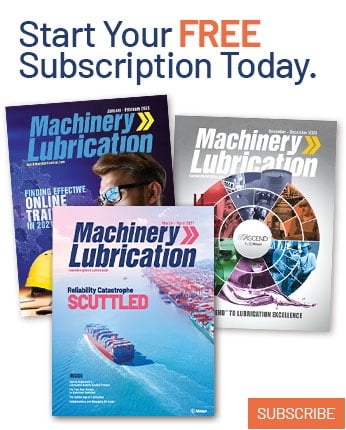